How do marine creatures and submarines not crumple under the weight of the ocean?
It's a PRESSING question.
You got friends, nerd? I bet you’ve got all kinds of friends. A bunch of really great, nerdy friends who’d probably love to read about some deep-sea anti-crumple (that’s the scientific term) technology and the literal weight of the planet. Maybe send this post to them when you’re done here. I think they’d like that.
I’ll put another button at the bottom so you don’t have to scroll all the way back up. You’re welcome (and thank you)!
Crushing it.
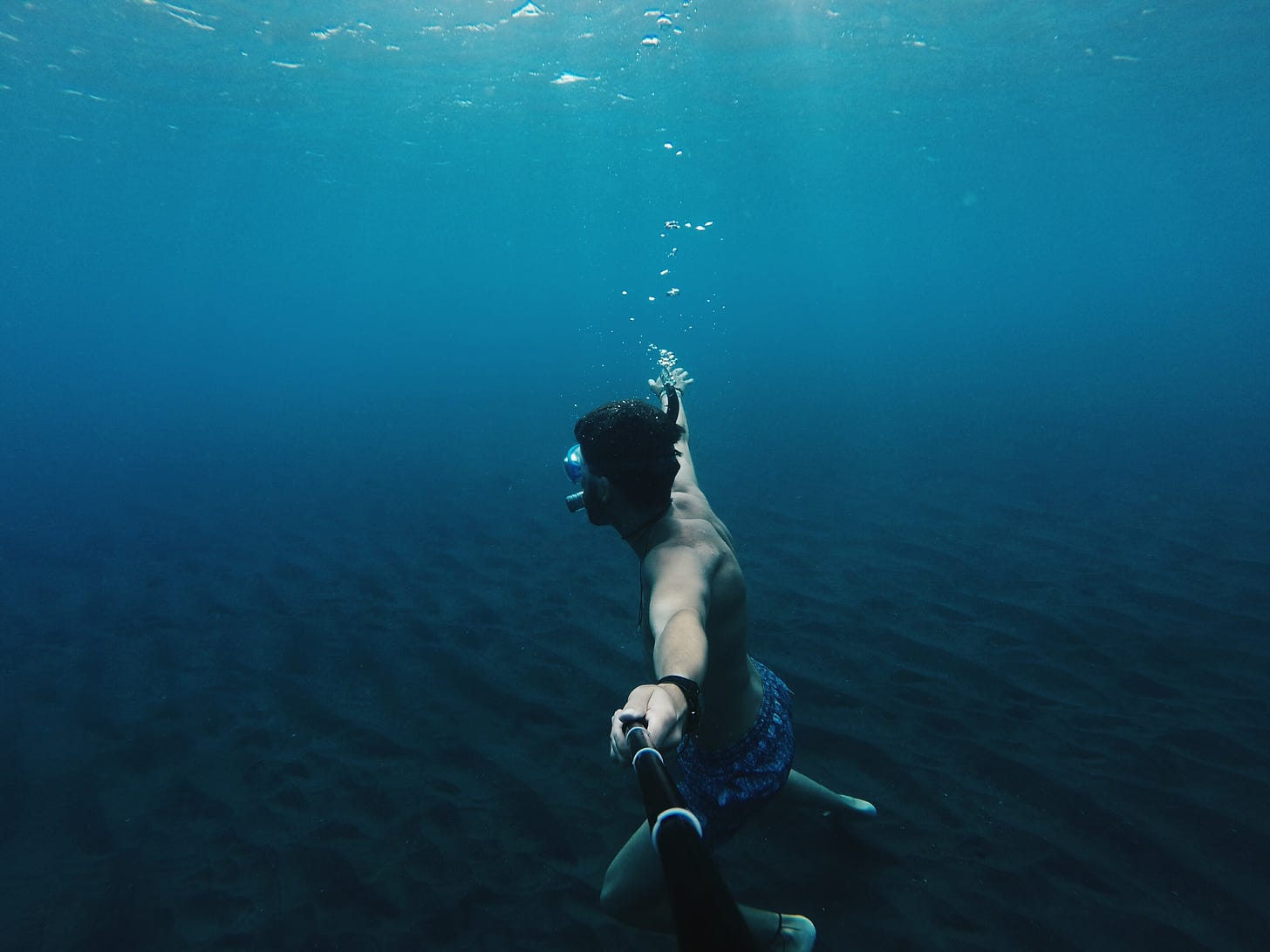
You know how if you’re at sea level, there’s a measure of air pressure? Right. Based on their relative position to the surface of the Earth, air particles behave differently because of where they are. The ones closest to the ground get squashed down together by the weight of the the ones above and become a super dense layer of air. That’s why it’s easier to breathe the closer you get to sea level. There are just more air molecules in every breath.
The higher up you go, the less atmosphere there is to exert pressure around you and those same air molecules float further and further apart. Less air in every breath, less pressure on the things that are up there. (Remember Col. Joe Kittinger’s balloon hand? Nothing to press down on it.)
The Earth’s atmosphere is about 122 km thick and weighs over 5.5 quadrillion (!!!) tons, about one millionth of the planet’s mass. Spread over the entire surface, it’s the equivalent of about 14.7 lbs (6.67 kg) of pressure per square inch pressing down on us. We don’t actually feel it because the ambient pressure inside our bodies is the same, so they even out. (even more insight into the balloon hand! More pressure pushing out from the body than pushing down by the air!)
That baseline pressure is called an atmosphere. At sea level, the measurement is 1 atm*, which quickly rises based on underwater depth. Underwater, the pressure increases by an atm every 10.06 metres; by the time a sperm whale reaches depths of 2 km or more to hunt for giant squid, the pressure reaches over 200 atmospheres, an equivalent of 2940 psi. No thanks!
* Barometric pressure is the more common measurement, but I like the drama and intrigue of referring to atmospheres’ worth of pressure. It actually becomes more helpful for demonstrating just how pressurized deep ocean environments actually are in a tangible way if psi isn’t your thing.
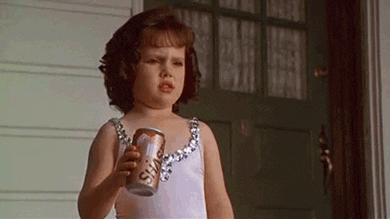
Going higher in elevation will see the atm measurement slowly decrease over the hundreds of kilometres between us and the vacuum of space. Dipping below the ocean’s surface, though, the atmospheres rise rapidly and become a significant source of trouble for most life forms almost immediately.
[The next section has a fairly detailed description of what happens to living things when their bodies encounter highly pressurized underwater environments. Scroll down to the ocean picture to skip it!]
Since we explored what happens to most living things when they’re exposed to a vacuum [thanks again, Col. Kittinger], it’s a little easier to imagine the opposite.
Hundreds of atmospheres’ worth of pressure crushes just about everything to bits, especially if they have air stored inside (lungs, oxygen tanks, most of the things that support land-dwelling life), since air is highly compressible and there are very few materials that can withstand that kind of environment.
No surprise to anyone, humans can’t last long past a hundred feet down or so.
At a depth of around 100 feet, (remember, you’d have four times the normal pressure pushing down on you at this point), the spongy tissue of the lung begins to contract, which would leave you with only a small supply of air that was inhaled at the surface. An ancient “dive-response” is then triggered in our body, which constricts the limbs and pushes blood toward the needier heart and brain. This extra blood expands the blood vessels in the chest, which balances out the pressure from the outside water.
During their deepest dives, a diver’s heart rate can dip to only 14 beats per minute; for reference, this is about a third of the rate of a person in a coma. [O]ur instinct to survive can do some pretty [wild] things at life-or-death moments. A convenient mechanism, for sure, but we can’t survive like this for long.
At a deep enough level [likely at about the 400 foot mark], the lungs would collapse completely, killing you instantly.
For land lubbers, the ocean poses a few other risks before we even have to worry about being crushed by the ocean itself. Since our bodies carry both the oxygen and nitrogen we breathe in the bloodstream, added pressure on arteries and veins encourage the nitrogen to be absorbed. Not good. This is what’s been dubbed the Martini effect.
The high partial pressures of nitrogen experienced by divers on deeper dives has an anesthetic effect on the brain, it can cause a sense of euphoria, impair motor abilities and coordination, lead to poor judgment and reasoning, and in extreme cases, prevent the diver from remembering much of the dive.
For every atmosphere of pressure, the extra nitrogen applies about the same level of “inebriation” as about one drink. Nitrogen narcosis isn’t just feeling tipsy underwater; it’s led to divers taking unbelievable risks or losing consciousness.
At about 100 feet the pressure will cause enough nitrogen to dissolve in the blood for the nitrogen to become a danger.
Before the stupor stage, divers become dizzy, their ability to make even simple mental decisions [like tell time or react to emergencies] is reduced. Sometimes they decide they no longer need to breathe through their mouthpiece.
So any air in the body equals incapacitation, if not death, while the weight of the ocean presses down at an insurmountable level. For more info on “barotrauma,” what happens to people during extreme swings in barometric pressure, check this out.
Well before we’re even suffered the effects of the pressure, humans just aren’t set up to survive the deep. Any air in the body, even if it’s in its own cavity (inner ear, sinuses) or surrounded by a bone cage (a pretty metal name for ribs, no?), gets mashed. All the life that exists down there has evolved well past the limitations of land-dwellers.
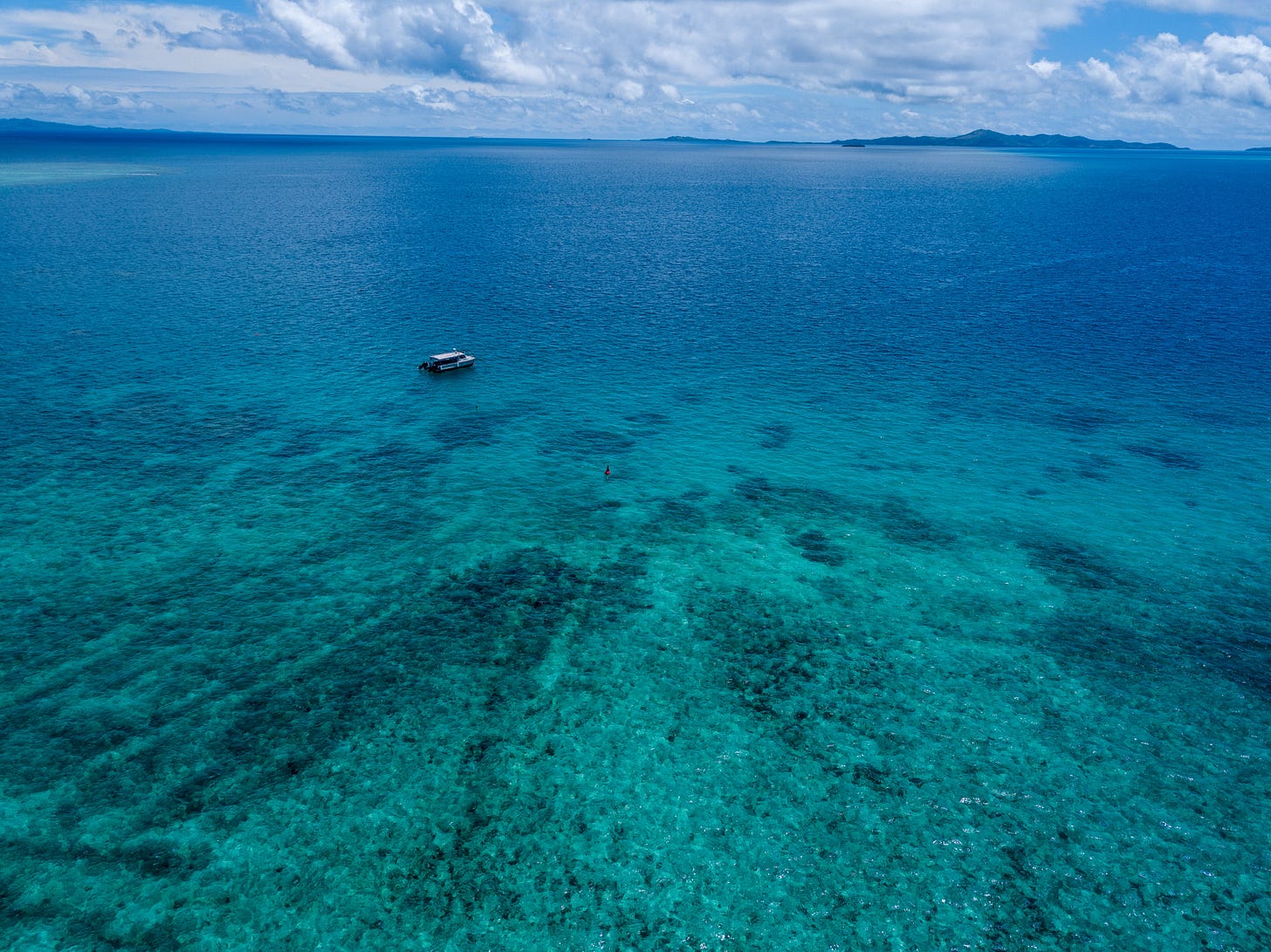
[It’s over! Read on for evolutionary characteristics of whales!]
According to the U.S. National Oceanic and Atmospheric Administration, deep-water species can survive at such great depths because (drumroll) their bodies adapted to it!
Whales, for instance, can withstand dramatic pressure changes because their bodies are more flexible. Their ribs are bound by loose, bendable cartilage, which allows the rib cage to collapse at pressures that would easily snap our bones.
A whale's lungs can also collapse safely under pressure, which keeps them from rupturing. This allows sperm whales to hunt for giant squid at depths of 7,000 feet or more.
Even in cases where whales dive well past the threshold of using the oxygen stored in their lungs, they’ve adapted to store oxygen in their muscles and blood and can absorb it from there for up to two hours at depth. There’s no risk of rupture when oxygen can be stored outside the lungs in less compressible liquids and tissue.
In the same way that whales’ bendiness allows them to dive freely, the same can be said for the airless, water-rich bodies of squid, octopi, clams, fish, and sea cucumbers who can live in perfect comfort under thousands of atmospheres of pressure.
If we revisit grade school science once again, you might recall that gases are fairly compressible (think of an empty plastic water bottle, then think about how it feels to squeeze - it crumples a bit, right?) since there’s more space between the molecules.
Liquids, on the other hand, not so much. They’re virtually incompressible. It makes so much sense that the creatures with the least gaseous or porous elements and the most liquid components to their bodies have the best chances to live in the deep.
So how the heck can submersibles possibly reach the ocean’s coldest, darkest, weirdest depths, since they break literally all of the deep-sea survival rules there are?
Engineers definitely took some evolutionary queues from marine life to make their technology feasible, but they came through with some seriously creative concepts to allow humans and machinery to function under pressure.
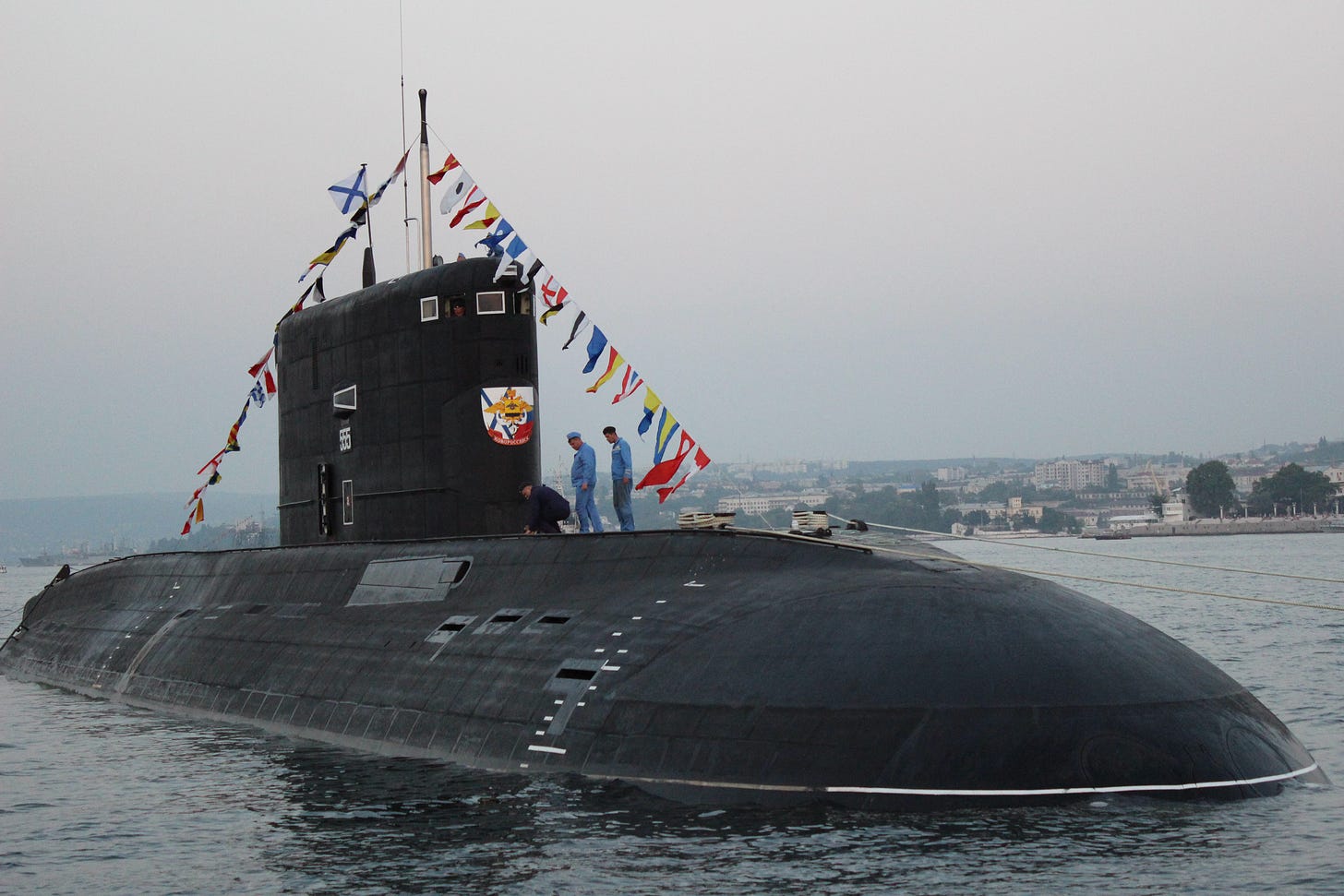
Man, did I ever learn some wild stuff about submarines for this post! It turns out most military subs don’t actually dive that deep, usually to a maximum of about 800m or so. A few scarce Soviet models had test depths of over 1000m, but since military subs have so much specialized technology on board that would succumb to severe stress under pressure, most of the deepest-diving submersibles are civilian bathyscaphes.
Typically, military submarines are double-hulled. The outer hull is thin and acts as the first line of defence against atmospheric pressure; its steel construction is actually designed to expand and contract a bit to absorb some of the effects before they reach the inner pressure hull. It can also take damage and disperse impact away from the vessel without impacting the sub’s ability to resurface as long as the pressure hull is still intact.
The pressure hull is waaaaaay stronger than the outer hull and is constructed to fully withstand the difference in pressurized environment between the livable interior and the totally deadly exterior. A series of complex structures built around the hull reinforces it so it can withstand the pressures of the deep, and the measurements have to be perfect for it to work:
Even a one-inch (25 mm) deviation from cross-sectional roundness results in over 30 percent decrease of hydrostatic load.[3] Minor deviations are resisted by the stiffener rings, and the total pressure force of several million longitudinally-oriented tons must be distributed evenly over the hull by using a hull with a circular cross section.
This design is the most resistant to compressive stress and without it no material could resist water pressure at submarine depths. A submarine hull requires expensive transversal construction, with stiffener rings located more frequently than the longitudinals. No hull parts may contain defects, and all welded joints are checked several times with different methods.
The rings and joints really do most of the heavy lifting here.
What really struck me as super cool and unexpected was the way that bathyscaphes survive the deepest of the deep. If military subs haven’t make the dive into Challenger Deep, how could one of these?
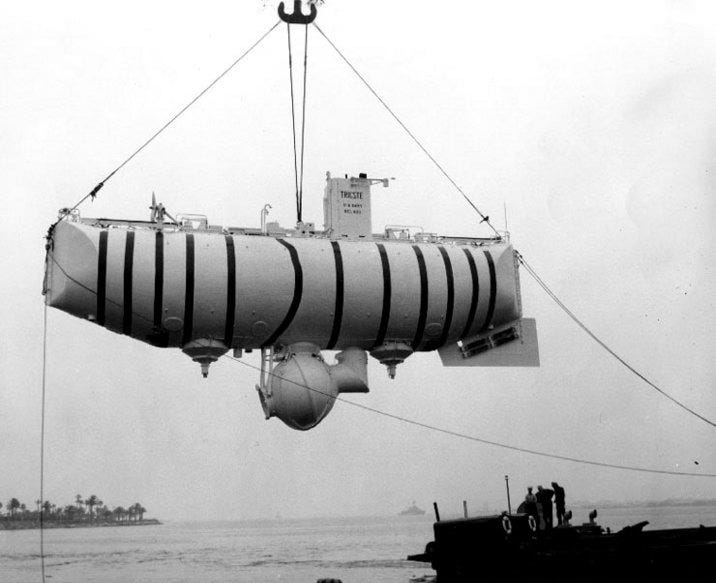
It turns out that the limitations of regular submarines is around the need to use compressed air to refill ballast tanks when it’s time to ascend. (Subs typically take on seawater as ballast to descend.) At a certain depth it’s no longer helpful to try to use air to force water out of the tanks because they’d just collapse.
In order to offset the weight of the onboard seawater without the use of air, bathyscaphes have done two things:
One, they filled the main buoyancy capsule with gasoline, since it’s more buoyant than water and even more compression-resistant, so it works double duty to prevent crush damage and aids in the ascent.
And two, they used iron shot for displacement. Since it’s more dense than water, ‘scaphes dive with the iron already onboard and eject it onto the ocean floor from its own electromagnetically-controlled container. Even in the event of power loss the shot is released automatically, increasing the vessel’s buoyancy and returning it to the surface with the help of the floaty gasoline.
Obviously there are big developments here around the material strength, too. I mean, you can’t just throw a submersible down 11,000m (that gives me the heebs just thinking about it) and expect materials to just stay functional. Or can you???
It was a huge surprise to learn that the most significant differences between the Challenger Deep-bound bathyscaphe Trieste and other subs, outside of the use of gasoline to offset the need for super reinforced materials, are just the thickness of its walls.
So incompressible gasoline protected the major part of the Trieste from compression, but what about the pod with the live humans in it? They still need air to breathe for the journey.
It turns out it was an exercise in sheer willpower on the part of the designers. The human module of the Trieste (see that balloon-looking thing attached to the bottom of the vessel?) had walls 12.7 cm (5.0 in) thick, plus a tiny cone-shaped plexiglass window for observation, each strong enough to withstand 1.25 metric tons per square centimetre. I guess brute force works sometimes? Even so, that’s…a lot of atmospheres.
Here are the first two humans (and the first human voyage of only two) to have seen the lowest point on Earth, Challenger Deep.
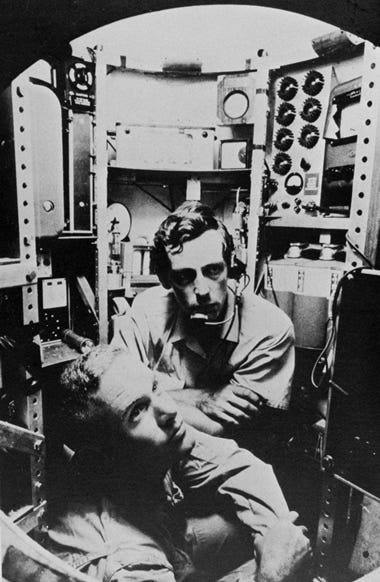
Don Walsh and Jacques Piccard ventured down in the Trieste in 1960 and got to see the most extreme environment on this planet for themselves. According to their log, they saw flatfish resembling sole or flounder on their way down, which totally tracks.
Got thoughts and feelings about the ocean? Let me hear ‘em!
Here’s that share button we talked about. Just imagine it’s slightly squashed under the weight of this post, that’ll make it fun.